Effect Of The Antarctic Continent On Atmospheric Circulation In The Southern Ocean
The term "katabatic" (after Greek for "go down") refers to a wind caused by the local descent of cold air down a slope. In the Antarctic, katabatic winds are a large-scale phenomenon, similar to trade winds in the magnitude of energy transfer (Weller, 1982). A highice plateau, coupled with low cloud cover, permits intense radiational cooling near the ground, and this sets up strong surface "inversions" (i.e., air temperatures increase with height, which is the reverse of the normal situation in the lower atmosphere). Having the coldest air closest to theground meansan atmospheric column is very stable to vertical motions, and soconvection is严重的限制。然而,冷空气可以流失off the plateau down to the coastal regions, with the Coriolis force generating a westward component of motion. With topography playing such a large role in the surface wind regime, the directionality of the flow is often remarkably constant. Some locations are more prone than others to katabatic winds. Cape Denison, site of Sir Douglas Mawson's 1911-14 Australasian Antarctic Expedition, is probably the windiest place on Earth and inspired "The Home of the Blizzard" (Mawson, 1915). According to Mawson, winds of 100 miles per hour (about 45 m.s"1) were frequent and, on one occasion,wind speedsof over 200 miles per hour were reported.
In terms of atmospheric dynamics, it is necessary to distinguish between the persistent surface winds (often called "inversion winds") over the gently sloping Antarctic interior, and the unusually strong katabatic winds near the steep coastal escarpment (Schwerdtfeger, 1970; Parish, 1982). The continental inversion winds (of about 2-10 m.s-1) can be viewed as an equilibrium flow balancing the sloped-inversionpressure gradient force(由地形)和Coriolis and frictional forces. The strong coastal phenomena, where wind speeds can jump almost instantaneously from calm to 15-20 m.s4, are topographically forced patterns of cold air convergence. Katabatic wind flows in other parts of the world are characterized by short lifetimes, as the upstream reservoir of cold air is rapidly depleted. In Antarctica, however, the convergence in thecontinental interiorsupplies some coastal sites, such as the Cape Denison region in Adelie Land, with a virtually inexhaustible supply of cold air.
Air draining off the Antarctic continent is turned westward by the Coriolis force to produce the persistenteasterlieson the poleward side of the Circumpolar Trough. Other effects of coastal katabatic flows, such as the extreme gustiness and blowing snow of a "katabatic storm", do not extend more than a few kilometres offshore. The persistent winds can also agitate surface waters and prevent ice formation, as has been observed at Terra Nova Bay 300 km north of theRoss Ice Shelf(Bromwich and Kurtz, 1982).
Numerical Simulations
Antarctica has a marked topographic and thermal effect on the large-scale atmospheric circulation over the Southern Ocean. Standing-wave patterns in the atmosphere are greatly influenced by mountain barriers and by heat sources and sinks. The continent acts as an elevated heat sink, and the asymmetry of Antarctica about the Pole produces a corresponding asymmetry in the sea surface temperature distribution in high latitudes. At 55°S, for example, surface water temperatures in the South Atlantic and South Indian Oceans are typically about 5°C colder than in the South Pacific Ocean. There are also significant differences between the middle and high latitude circulations of the Northern and Southern Hemispheres, which are in part due to the different land-sea configuration near the poles. In the Southern Hemisphere,the westerliesare stronger and the polar trough much more intense and persistent. It has been suggested (e.g., Mechoso, 1981) that the persistence of the Antarctic Circumpolar Trough results from the Antarctic plateau presenting a physical barrier to the southward movement of cyclones, causing them to cluster along theice edge。If this were the case, one might expect a rather different sea level pressure pattern if Antarctica were removed. This supposition can readily be tested by experiments with numerical models of the global atmosphere.
Such "general circulation" or "climate" models solve a set of equations describing basic physical laws (such as the second law of thermodynamics, and conservation laws for momentum and water vapour). A detailed treatment is made of the fluxes of solar and infrared radiation through the atmosphere, and the various interactions which can occur at the atmosphere-surface boundary. Because of the complexity of the overall system, it is common for some simplifying assumptions to be made. Usually, at least one of the parameters defining cloudiness, sea-surface temperature or sea ice amount is specified at observed values (rather than allow the model to calculate its own values). Modelling of the oceanic circulation has not yet reached the stage where a coupled atmosphere-ocean climate model can predict ocean heat transports with any confidence. A standard approach is therefore to couple theatmosphere modelto a much simpler model of the ocean in which seasonal heat storage (i.e., varying sea-surface temperature) is allowed but no horizontal transport of heat occurs. Unfortunately, neglecting oceanic heat transport in this way tends to produce lower temperatures and more sea ice in high latitudes than observed.
Model calculations of theglobal atmospheric circulationthus have a number of shortcomings, and the errors tend to be particularly large in polar latitudes. Most climate models underestimate both the strength of the westerlies in the Southern Hemisphere and the intensity of the Antarctic Circumpolar Trough. Herman and Johnson (1980) reported on a "grid-point" climate model which was unable to reproduce the stationary low pressure centres around Antarctica, with the predicted pressures in the Circumpolar Trough being typically 20 hPa too high. "Spectral" models, which did not come into general use until the late 1970s, adopt a different mathematical technique to solve thegoverning equations, and tend to give a more realistic high latitude simulation than grid-point models. In spectral models, the dynamic variables (e.g., pressure, temperature, wind) are represented by a sum of spherical harmonics, in contrast to the grid-point approach where the variables take values on a network of discrete points. The geometrical formulation of the spectral approach is ideally suited to solving equations on a sphere, and means that spatial derivatives can be evaluated exactly. This is a particularly important advantage in simulating high southern latitude circulation since sharp gradients in many variables occur around the Antarctic escarpment. Manabe et al. (1978) showed that the latitudinal distribution of sea-level pressure, distinctly different in the two polar regions, was reproduced quite well by their spectral model (Fig. 2.17). Increasing the model resolution (i.e., including higher harmonics) strengthened the mid-latitude westerlies in the winter hemisphere, which generally improves the simulation for the Southern Hemisphere but not for the Northern Hemisphere. The January simulation (not shown) had the Antarctic
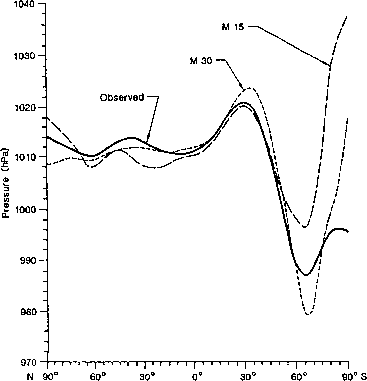
Latitude
Fig. 2.17. Latitudinal variation of the July zonal mean sea level pressure (in hPa) as observed (solid line), and as simulated by the Geophysics Fluid Dynamics Laboratory spectral model. Two simulations are shown, one at low resolution (M15) and another at high resolution (M30) (from Manabe et al., 1978).
Latitude
Fig. 2.17. Latitudinal variation of the July zonal mean sea level pressure (in hPa) as observed (solid line), and as simulated by the Geophysics Fluid Dynamics Laboratory spectral model. Two simulations are shown, one at low resolution (M15) and another at high resolution (M30) (from Manabe et al., 1978).
Circumpolar Trough too weak by about 8 hPa at all resolutions and the Northern Hemisphere polar trough much too intense.
Bearing in mind the above comments about the systematic errors in the models, we now consider two experiments which attempt to highlight possible effects of Antarctica on high latitude circulation. Mechoso (1981) examined topographic influences on the Southern Hemisphere general circulation by comparing a "control" circulation with an "anomaly" circulation where all topography in the Southern Hemisphere was reduced to zero. The simulation was for the month of July and used the spectral model of Manabe et al. (1978) referred to above, whose control simulation was known to be fairly realistic. In the absence of all mountains (the "anomaly" run), the high latitude surface westerlies were weaker and extended further poleward. The areal extent of the surface easterlies was much reduced, with the axis of the Circumpolar Trough being near 80°S over continental "Antarctica". Depressions which formed in mid-latitudes still followed a general south-easterly track, but no longer showed the clustering around the Antarctic coast. Very little high latitudecyclogenesisoccurred in the no-mountain case as compared to the control run. In the absence of Antarctica, the atmospheric standing waves in high latitudes were also much weaker, with their phases unrelated to observations, in spite of the model prescribing the observed sea-surface temperature pattern.
Simmonds and Lin (1983) used the Australian Numerical Meteorology Research Centre spectral model, and compared a January control simulation with the circulation in three anomaly experiments: no mountains anywhere in the Southern Hemisphere, the removal of just Antarctica (replacing it by sea at -1°C), and the imposition of a longitudinally uniform sea-surface temperature. The control simulation had the common failing of a Circumpolar Trough substantially weaker than observed. Rather surprisingly, the topographic anomaly experiments showed little significant change in the surface pressure field — the subtropical highs and Circumpolar Trough were present in all runs, in much the same location. Larger changes were noted at upper levels, where the intensity of the standing-wave pattern was much reduced. The removal of Antarctica affected the 500 hPa height field as far north as 45°S. The zonally uniform sea-surface temperature run produced little effect in high latitudes, but thermal forcing was shown to be the dominating influence in locating the subtropical oceanic highs on the eastern sides ofthe ocean basins。尽管Mechoso(1981)和西蒙兹和林(1983) reached somewhat different conclusions, they both showed that the presence of Antarctica can have effects on the circulation of the Southern Hemisphere far away from the continent itself.
Continue reading here:Summary
Was this article helpful?
Readers' Questions
-
Ottavia1 year ago
- Reply