Stabilization Ponds
One of the ancient wastewater treatment technologies, the stabilization pond (also referred to asa lagoon), has been used continuously as a method of sewage disposal. In some cases, these ponds were also utilized foraquaculture. Stabilization ponds are used for both municipal waste-water treatmentandindustrial wastewater treatment, particularly for wastewaters from small communities and seasonal industrial wastewaters as well as less affluent communities throughout the world (Fig. 6.1). Although stabilization ponds can be used in most regions of human habitation, their performances in treating wastes are at best in warm climates with adequate sunlight. The current interest inwaste stabilization ponds(wsp)是一个意外的发现的结果their capabilities when WSPs were used initially as simple sedimentation basins or emergence holding ponds at wastewater treatment plants. A WSP is a relatively shallow body of wastewater contained in an earthen man-made basin into which wastewater flows and from which, after a certain retention time (time that takes the effluent to flow from the inlet to the outlet), a well-treated effluent is discharged. Many characteristics make WSPs substantially different from other wastewater treatment. This includes design, construction and operation simplicity, cost effectiveness, low maintenance requirements,csgo雷竞技 requirements, easy adaptation for upgrading, and high efficiency. They are used for sewage treatment in temperate andtropical climates他们提供了一个最简单的,因为最低t, and most efficient wastewater treatment technologies available. Waste stabilization ponds are very effective in the removal of fecal coliform bacteria.Solar energyis the only requirement for its operation. WSPs have been in use in the United States since 1901. But until fairly recently, the United States Environmental Protection Agency (USEPA) has formalized the design and operational criteria for WSPs (USEPA, 1983).
Over 7,500 WSP systems have been built in the United States serving rural communities with a mean population of less than 10,000. In Canada, there are over 1,000 WSPs in operation representing about a half of the wastewater treatment capacity in the country (Townshend and Knoll,
-
- Figure 6.1. A waste stabilization pond system in Mèza, France. It contains two small anaerobic ponds, four experimental facultative ponds, and one series pond system composed of a facultative pond and four maturation ponds.
1987)。wsp是最常见的类型的废水treatment systems in many other countries; particularly in warmer climates (the Middle East, Africa, South Asia, and Latin America), ponds are commonly used for large populations (up to around 1 million). In developing countries and especially in the tropical and equatorial regions, sewage treatment by WSPs has been considered an ideal way of using natural processes to improve sewage effluents. In the recent decades, Europe has seen an increased number of WSPs built in many parts of the region where small populations reside (Gomes de Sousa, 1987). In France, there are approximately 2,500 WSPs in operation throughout the country, mostly for small communities (Racault et al., 1995). The capital and operational costs of WSPs are lower than other comparable wastewater treatment technologies (Mara and Pearson, 1998).
Waste stabilization ponds can be used alone or in combination with other wastewater treatment processes. A typical system consists of several constructed ponds operating in series; treatment of the wastewater occurs as constituents are removed by sedimentation or transformed by biological and chemical processes. In the bottom of the ponds, a sludge layer is formed, which can impact performance by changing the pond's hydraulics due to a decrease in the pond's effective volume and changes in the shape of the bottom surface. Therefore, periodic sludge removal is usually required. Stabilization ponds suitable for wastewater treatment are those that maintain the right biological conditions for biological interactions and reactions that break down the organic matters andinorganic nutrients. There are five basic types of WSPs. All use microorganisms to degrade and decontaminate organic and inorganic constituents; the types of organisms as well as the amount of oxygen present in the pond systems differ among the five categories forming the basis for classifying stabilization ponds:
• Maturation ponds
• Aerated ponds
• Aerobic ponds
The term oxidationpond isalso used to describe any pond system that utilizes oxygen to break down organic matters, either with or without a significant portion of the dissolved oxygen (DO) provided by photosynthetic algae. All stabilization ponds, with the exception of anaerobic ponds, can be grouped into theoxidation pondcategory.
Facultative ponds
The facultative ponds are the most common type of stabilization ponds in use and are able to completely treat both raw, settled sewage and a wide range of industrial wastewaters, including food and agricultural waste-waters with detention time of 5-30 days. These ponds have depth ranging from 1.2-1.5 m (4-8 ft), consisting oftwo layersof biological treatment zones: an aerobic layer on top of an anaerobic layer, often containing sludge. The aerobic layer stabilizes the wastewater while the fermentation takes place in the anaerobic layer. The oxygen needed for aerobic stabilization comes from photosynthesis of algae in the pond (Fig. 6.2). The algae are photosynthetic organisms and therefore provide a source of oxygen for use by heterotrophic bacteria in the pond for the aerobic breakdown of organic matter. Respiration of organic matter provides a source of carbon dioxide for the algae. This symbiotic relationship between the algae and the bacteria provides the basis for this natural method of waste-
光设置!
光设置!
-
- Anaerobic layer anaerobic bacteria-degradation
Figure 6.2. Aschematic diagramof a facultative pond with description of interactions among its components.
Figure 6.2.A schematic diagramof a facultative pond with description of interactions among its components.
water treatment. Because algae in the discharged effluent need to be removed, the treatment process usually involves a facultative pond, one or two maturation ponds and atertiary treatmentphase, such as rock filters or intermittentsand filtrationfor the removal of algae before discharge of the final effluent to an adjacent watercourse. Total containment ponds are suitable for climates where evaporativeloss of waterexceeds the rainfall; controlled discharged ponds are used in many areas and climates. The controlled discharged ponds operate discharges once or twice a year, depending on the quality of the treated water being discharged, and tend to have longer detention time.
The longer detention time and the issue of blooming algae in the treated water offset the savings associated with facultative ponds. An obvious solution to the inherited drawbacks of facultative ponds is to add oxygen to the ponds. In an aerated pond, oxygen is supplied to the pond through mechanical aeration equipment and air diffusers. The aerated ponds (which could still be classified either as facultative or as aerobic depending on their DO profile), unlike facultative ponds, can be built deeper, usually in the range of 2-6 m (6-20 ft) because oxygen can be introduced in lower depth. As a result, the detention time is shortened and is in the neighbor hood of 3-10 days. Depending on the way the oxygen is delivered to the pond, an aerated pond can be designed as a completely mixed or partiallymixed reactor, thus reducing the footprint of the pond. A completely mixed reactor used for an aerated pond has many similar design characteristics of an activated sludge reactor.
Maturation ponds
成熟的池塘,有时也称为tertiary-maturation ponds, are low-rate stabilization ponds designed to provide secondary effluent (conventional secondary processes or facultative ponds) polishing and pathogen removal. The mechanism for pathogen removal in maturation ponds is actually simple: the removal of microorganisms (pathogens) is due to natural die-off, predation, sedimentation, and adsorption. The majority of pathogens settle onto the sludge in the bottom of the ponds, thus are removed from effluents. In fact, all pond systems can, to some degree, remove pathogens from wastewaters. In order for maturation ponds to remove pathogens substantially, detention times in these ponds must be long enough for pathogens to settle down in the ponds. There is a risk if the sludge is removed from the maturation ponds; handling of the sludge requires caution and the sludge needs to be treated or kept out of the public access.
For high-strength wastewaters, maturation ponds are used for improving the effluent quality prior to surface water discharge of treated waste-waters. Their design, size, and number, in series, are decided in many parts of the world based on the need of removing pathogen from treated waste-water. If the objective of using maturation ponds is for maximum purity in terms of BOD5 reduction and pathogen removal, algae production is discouraged for maximumlight penetration. Maturation ponds, however, are of the same depth as facultative ponds (1 to 1.5 m or 3 to 4.5 ft). A minimum of 15 to 20 days is used as detention time for maturation ponds. Nitrogen removal is presented in these ponds through denitrification in the sludge settled in the ponds. Phosphorus may also be removed by diverse communities of algae. However, algal growth is not desired, particularly if the effluent form of maturation pond is slated to discharge into the receiving water because of turbidity and suspended solids caused by algae. Rock filters in submerged beds are used sometimes for removal of these algal solids. The algae retained by rock filters decompose and are utilized by bacterial biofilm on rock materials. In general, the arrangement of pond systems starts with an anaerobic pond, and then a facultative pond, and finally a maturation pond in a series, depending on the quality of the influent and the treatment objective.
Aerated lagoons
Anaerated lagoonis a stabilization pond with its aerobic condition maintained by mechanical or diffused aeration equipment. Bubble aeration is provided sometimes to keep the pond aerobic in locations where pond surfaces are frozen for extended periods in winter. Unlike aerobic ponds, aerated lagoons do not rely on algae for oxygen delivery. The microbial characteristics of an aerated pond are very similar to those of an activated sludge process. Its detention times are in the order of1 to 10 days, depending onorganic loading rate, temperature, and the degree of treatment required. The organic loading of aerated lagoons is expressed as BOD5 per unit volume per day. Aeration ponds are susceptible to a large amount of BOD5 loadings or有毒废物, which can severely hinder the ponds' efficiency. The solids in the pond need to be suspended all the time to avoid the solids settling in the bottom of the pond and forming an anaerobic layer, thus reducing the efficiency and generating odor. Fig. 6.3 shows a schematic portrait of an aerated lagoon and a photo of an aerator at work in an aerated pond.
Aerobic ponds
Aerobic ponds, also called high-rate aerobic ponds, are designed to maintain a constant dissolved oxygen (DO) level throughout the depth of the ponds. The aerobic ponds can be viewed as "turbo-charged" facultative ponds with added oxygen functioning. As aunit process, aerobic ponds fall between facultative ponds andactivated sludge processes. They are usually shallow (30-45 cm or 12-18 in), allowing light to penetrate the entire depth of the ponds. Oxygen is provided by both an external device and algae through photosynthesis; mixing is also provided to disperse oxygen and expose algae to sunlight as well as prevent anaerobic conditions in the ponds. The detention time for this type of pond is relatively short, about 3-5 days. In order to maintain the constant oxygen level in all depths, these ponds are best used in warm and sunny locations where photosynthesis of algae is quick enough to provide sufficient DO needed to degrade the organic matters in wastewater.
Mechanical surface aerator
Mechanical surface aerator
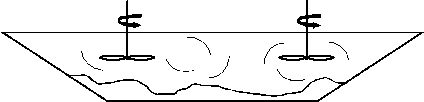
sediment
Figure 6.3. A schematic diagram of an aerated pond with surface aerators.
sediment
Figure 6.3. A schematic diagram of an aerated pond with surface aerators.
Anaerobic ponds
For strong food andagricultural wastewater, anaerobic ponds can be used to degrade the heavy loading of organic matters. In general, there are three identifiable zones in a basic design of an anaerobic lagoon: the scum layer, the supernatant layer, and the sludge layer. The system requires longer time (20-50 days); its depth is in the range of 2.5-5 meters (8-16.3 ft) and produces acid and methane. In anaerobic ponds, BOD5 removal is achieved by sedimentation of solids and subsequent anaerobic digestion in the resulting sludge. The process of anaerobic digestion is more intense at temperatures above 15°C. The anaerobic bacteria are usually sensitive to pH <6.2. Thus, acidic wastewater must be neutralized prior to its treatment in anaerobic ponds. A well-designed anaerobic pond will achieve about a 40% removal of BOD5 at 10°C, and more than 60% at 20°C. A shorter retention time of 1.0-1.5 days is commonly used. The design criteria foranaerobic lagoons是与众不同t from other designs of the stabilization ponds; the main noticeable difference is depth of the lagoon. Oxygen transfer through the air-water interface of a lagoon is not important at all; in fact, it is undesirable for the anaerobic lagoon. Thus, anaerobic lagoons are typically deep basins 2-5 meters (6.5-16.3 ft) in depth.
Anaerobic lagoons are often used as a preliminary treatment for high-strength wastewaters with high content of organic materials, such as those found in food processing wastewaters rich in fat and proteins. As a result, anaerobic lagoons can only partially stabilize wastewaters, and further treatment, most likelyaerobic processes, is needed before the wastewaters can be discharged to the receiving waters. It is common to place facultative ponds or activated sludge processes right after anaerobic lagoons for
-
- Figure 6.4. A photograph of an anaerobic pond for wastewater treatment in the field.
desired treatment goals. Slaughterhouses and meat processing wastewaters (BOD5: ~1,400 mg/l; FOG: ~500 mg/l; pH: ~7; temperature: 28°C) are particularly suitable for treatment by anaerobic lagoons after preliminary treatment such as removal of coarse solids and excessive grease or blood. Fig. 6.4 shows a picture of an anaerobic stabilization pond in the field.
Design of stabilization ponds
WSPs often comprise a single string of anaerobic, facultative or aerated/ aerobic, and maturation ponds in series, or several such series in parallel depending on the organic strength of influents and the effluent quality objectives. In essence, anaerobic and facultative or aerated/aerobic ponds are designed for removal of BOD, and maturation ponds for pathogen removal. For ease of maintenance and flexibility of operation, at least two trains of similar ponds in parallel are built in for many designs. High-strength wastewaters, with BOD5 concentration in excess of about 300
-
- Figure 6.5. Several stabilization pond configurations: A = anaerobic pond; F : facultative pond; M = maturation pond (adapted from Pescod and Mara, 1988).
mg/l, will frequently be introduced into first-stage anaerobic ponds, which achieve a high volumetric rate of removal. However, wherever anaerobic ponds are deemed unacceptable by the regulatory agencies or the public at large, wastewaters, regardless of strength, may be discharged directly into primary facultative ponds. Effluent from first-stage anaerobic ponds will overflow into secondary facultative ponds, which comprise the second-stage of biological treatment. Pescod and Mara (1988) summarized several common pond system configurations (Fig. 6.5), though other combinations may conceivably be used. The design loadings for stabilization ponds are usually measured in BOD per unit area. In anaerobic ponds, the loading is expressed as BOD per unit volume; this is because light, which is strongly affected by the surface area, is not an important factor in anaerobic ponds.
Facultative pond design is based onBOD removal, and most states set
Table 6.1. Recommendations for loading rates for variousclimatic conditions(adapted from Reed et al., 1995).
Climatic Condition BOD5 Loading Rate
Air temperature above 15°C in winter 45-90 kg/had (40-80 lb/acd)
Air temperature between 0 and 15°C in winter 22-45 kg/had (20-40 lb/acd) Air temperature below 0°C in winter 11-22 kg/had (10-20 lb/acd)
up their own design criteria for BOD loading and/or hydraulic detention time for facultative ponds. Reed et al. (1995) recommended the load rates for various climatic conditions for designing facultative ponds based on their experience (Table 6.1). Over the years, several empirical and rational models have been developed for the design of the ponds (Reed et al., 1995). These models range from ideal plug flow models to completely mixed pond models as well as something between these two extremes. Each of these models has produced satisfactory results in certain circumstances, but its use in the real world is limited because they require evaluating multiple coefficients, which have to be evaluated from the similar WSP systems. Reed et al. (1995) have compared several design models for facultative ponds and concluded that there was no "best procedure" for recommendation in designing facultative ponds.
The BOD loading rate in the first cell is limited to 40 kg/ha-d or less, and the total hydraulic detention time in the system is 120-180 days when the average temperature is below 0°C; the loading can be increased to 100 kg/ha-d when the average temperature is above 15°C (Reed et al., 1995).
The size of facultative ponds
The size of facultative ponds is determined by the following (Equation 6.1):
where:
Ls = surface BOD5 loading, kg/ha-d Li = influent BOD5, mg/l Q = flow, m3/d
Af = facultativepond area, m2
Table 6.2. BOD5 reduction as a function of detention time and temperature (adapted from WHO, 1987).
Temperature (°C) Detention Time (d) BOD5 Removal Rate (%)
10 5 0-10
10-15 4-5 30-40
15-20 2-3 40-50
20-25 1-2 40-60
25-30 1-2 60-80
There is no consensus when it comes to the best design approach for anaerobic ponds. Customarily, volumetric BOD5 loading rate, temperature, and hydraulic detention time form the basis for anaerobic ponds. The World Health Organization (WHO) recommends the following guidelines for BOD removal rate 50% or higher (WHO, 1987):
• Volumetric loading, Lv, up to 300 g BOD5 kg/m3-d
• Hydraulic detention time of about 5 days
Table 6.2 shows BOD5 reduction as a function of detention time and temperature (adapted from WHO, 1987).
The size of anaerobic ponds
Anaerobic ponds can be satisfactorily designed, and without risk of odor nuisance, on the basis of volumetric BOD5 loading (lv, g/m3d), which is given by the following (Equation 6.2):
Lv = volumetric BOD5 loading, kg/m3-d or lb/ft3-d Li = influent BOD5, mg/l Q = flow, m3/d or ft3/d Va = anaerobic pond volume, m3 or ft3
Hydraulic balance
To maintain the liquid level in the ponds, the inflow must be, at least, greater than net evaporation and seepage at all times. Thus, we use the following (Equation 6.3):
where:
Qi = inflow to first pond, m3/d or ft3/d A = total area of pond series, m2 or ft2
e = net evaporation (i.e., evaporationless rainfall), mm/d or in/d s = seepage, mm/d or in/d
许多防渗设计用于处理BOD5和TSSloadings, and their capacity of removing BOD5 and TSS is well documented and reasonably representative in their designs; however, nitrogen removal capacity of these ponds is crucial given the fact that food and agricultural wastewaters tend to be rich in nitrogen content and that ammonia in treated waste-waters can be detrimental to fishes in the receiving waters. And in the case of sludge from pond systems that is used inland treatment, nitrogen in the sludge may affect the design of the land application and its costs. There are two importantempirical modelsdeveloped based on the real data from the fields and they are validated by the data in later studies. These two models are summarized in Table 6.3 and Table 6.4.
Phosphorus removal in stabilization ponds is an important design consideration only in north central United States and Canada. For example, the discharge limit for phosphorus in the Great Lakes is 1 mg/l. In order to achieve this criterion, chemicals are added to the pond system to reduce the phosphorus level. A typical alum dosage of 150 mg/l may reduce phosphorus to less than 1 mg/l and less than 20 mg/l BOD5 and TSS (Reed et al., 1995).
Without chemical addition, phosphorus removal in stabilization ponds can still be achieved. The mechanisms of phosphorus removal most likely take place in maturation ponds (Mara et al., 1992). The efficiency of total phosphorus removal in WSPs depends on how much biomass settled in the bottom of the pond systems; the biomass utilizes the phosphorus in waste-water (as described inbiological phosphorus removalin Chapter 5). Thus, the best way of increasing phosphorus removal in WSPs is to increase the
Table 6.3. Design model for nitrogen removal from stabilization pond systems (Pano and Middlebrooks, 1982).
For temperatures below 20°C:
Ce = Q / {1 + [(A / Q) (0.0038 + 0.000134T) exp ((1.041 + 0.044T) (pH - 6.6))]} For temperatures above 20°C:
Ce = Q/ {1 + [5.035 X 10-3 (A / Q)] [exp (1.540 X (pH - 6.6))]} Where:
Ce = ammonia-N concentration in pond effluent, mg N/l
Ci = ammonia-N concentration in pond influent, mg N/l
Q = influent flow rate, m3/d pH = 7.3 exp(0.0005 Ai)
Ai= influent alkalinity, mg CaCO3/l
Table 6.4. Design model for nitrogen removal from stabilization pond systems (Reed, 1984).
Ce = Ci exp{-[0.0064 (1.039)T-20] [q + 60.6 (pH - 6.6)]} Where:
Ce = total nitrogen concentration in pond effluent, mg N/l
Ci = total nitrogen concentration in pond influent, mg N/l
Aj= influent alkalinity, mg CaCO3/l number of maturation ponds so that, little by little, phosphorus becomes immobilized in the sediments. For a well running two-pond system, 70% mass removal of total phosphorus may be achievable.
Continue reading here:Land Treatment Systems
Was this article helpful?
Readers' Questions
-
VALENTINO4 months ago
- Reply
-
ROSA4 months ago
- Reply
-
Impi5 months ago
- Reply
-
abela7 months ago
- Reply
-
Petri1 year ago
- Reply
-
MICHAEL1 year ago
- Reply
-
Aamos1 year ago
- Reply
-
daisy1 year ago
- Reply